BIOME.cc – Biome evolution and climate change
Capucine Lechartre, Haozhi Ma, Elena Plekhanova, Manuel Richard Popp, Gengchen Yang
2024 - 2028
Cooperation- Federal University of Goiás, Brazil
- Helmholtz Zentrum für Umweltforschung
- Michigan State University, East Lansing, MI, USA
- National Aeronautics and Space Administration NASA
- Ohio State University, Columbus, OH, USA
- Peking University, Beijing, China
- University of Otago, Neuseeland
- University of Calgary, Canada
- USDA Forest Service, Missoula, MT, USA

Terrestrial ecosystems show evidence of increasing stress symptoms in response to the progressing climate change. In this project, we combine a global analysis of ecosystem disturbances with a new biome theory to more precisely forecast where on earth what types of disturbances may surface in response to ongoing climate change. Testing a new biome theory will additionally allow an improved understanding of how biomes have evolved and how global biodiversity has emerged and got spatially structured.
Global Biomes ¶
The progressing climate change is constantly changing the spatial distribution of biodiversity. We know relatively well how single species are and will respond to climate change. Yet, we understand much less well how whole ecosystems and global vegetation zones (biomes) respond to climate change. Global biomes represent structurally and physiogenomically uniform vegetation zones, which can also be separated climatically well. Examples are the “tropical rainforest”, the “boreal needleleaf forest”, or the “tundra” (Fig. 1). The structural uniformity of biomes has emerged as a result of convergent evolution of similar plant form and traits of biome-dominant plants consistently in similar climate across the globe and irrespective of the evolutionary heritage of the species. These biome-typical functional properties stand for an optimal adaptation to the prevailing climate and allows these plants to dominate under these conditions. A biome occupies a relatively large area on the globe and can usually be found on several continents. Within a biome, the climate varies and so does the species composition; yet as long as the biome climate is not exceeded, the dominant plant species have the same structural and functional properties. These properties are the result of plant evolution over millions of years. Species of the tropical rain forests in South America, Africa and Asia are barely related to each other, their evolutionary pathways and heritage are too different. Yet plant evolution has nevertheless led to very similar structures in all three regions under similar climates; we call this convergent evolution.
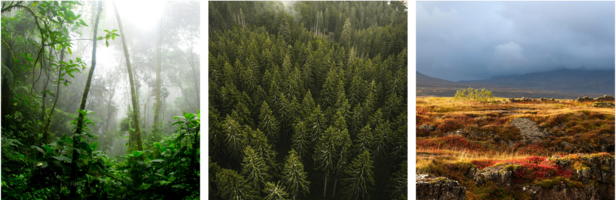
Goals of the research project ¶
For this project we investigate two related goals. The first goal aims at exploring and testing a new biome theory, the biome size – stress resistance theory, to better understand how biomes have evolved through evolutionary processes and how this has resulted in the current structure and distribution of global biodiversity. The second goal aims at assessing and projecting ecosystem disturbances in response to ongoing and future climate change related to biome shifts.
1) The biome size – stress resistance theory ¶
Biomes have evolved over millions of years of convergent evolution under similar climates on different continents. However, the area of biome patches on continents are not identical; neither spatially nor temporally. This means, that through time, a biome has and always had a different and changing area available within which plant evolution has taken place. Also, there may have been different patches of the same biome on one continent, but these patches were variably well connected to each other. Evolutionary biology has formulated “biome conservatism theory”, positing that biomes represent evolutionary arenas within which evolution was more or less spatially constrained and meaning that most species that have evolved have remained within the same biome. If this is true then the varying spatial dimension and connectedness of biome patches through time will have resulted in differing species richness per biome per continent, simply following the “island biogeography theory”. And if true, then differing richness between patches of the same biome can be expected to result in fitness differences to resist abiotic and biotic stress.
Therefore, we here formulate the “biome size – stress resistance” theory, which predicts that more biodiverse biome patches exhibit a higher tolerance of the biome-dominant species to abiotic (e.g., for cold or drought) or biotic (e.g., biological invasions) stress. Practically, this means that if a biome has a small area on continent “A”, it is less tolerant to abiotic or biotic stress tolerance than if the same biome has a large area on continent “B”. Plausible examples are the high rates of biological invasions (biotic stress) on small and/or long disconnected islands (Hawai’i, New Zealand) or continents (Australia) or the tree line temperature on continental (well-connected) vs. oceanic (disconnected) islands (Fig. 2). And the term area stands for the integral of area and connectedness, now and through the last 5-10(-20) million years relevant to evolve the contemporary plant biodiversity- To test this theory, we formulate several analyses steps.

Analysis steps
- Defining global biomes uniquely from functional and structural properties without using climate properties
- Mapping global biomes from functional and structural properties
- Testing the biome conservatism theory for different global biomes
- Assessing the area and connectedness of past biome patches (last 10-20 million years using BIOME4)
- Assessing the evolution of plant diversity with or without assuming biome conservatism (+/- strict evolutionary boundaries between biomes) for the last 10-20 million years using Gen3sis and comparing the simulation results with biome-wise plant richness compilations from global databases and models
- Identifying climate boundaries of mapped biome patches on different continents (from step 2) to test the formulate theory.
We currently define (1) and map (2) biomes globally and start analyzing the biome conservatism theory for different biomes, by both compiling data from the literature and by analyzing phylogenies of biome-dominant plant clades (3). Further, we d) start exploring the area and connectedness of biome patches using the BIOME4 dynamic process model over the last 10-20 million years (4). Based on this information, we will later simulate different scenarios of plant evolution by assuming more or less strict evolutionary boundaries between biome patches over the last 10-20 million years (5). During this period, the majority of extant plant species has evolved and is therefore relevant for testing our “biome size – stress resistance theory”. Climatic boundaries of mapped biomes are identified in connection with the second goal (6).
2) Ecosystem disturbances from climate-change related potential biome shift ¶
Over the past 40 years, we have seen a dramatic rate of rapidly increasing global temperatures and associated regional changes in precipitation patterns. While these changes have shifted the potential geographical boundaries of all major biomes of the globe, the actual shifts of biomes lag severely. This is due to the fact that shifting thousands of plants, animals, fungi and microbes takes decades, centuries and millennia. We therefore have many regions of individual biome patches that are no longer in sync with their climate (Fig. 3). This can lead to severe abiotic (drought, fire, windthrow) or biotic (pest outbreaks, biological invasions) disturbances. We can further expect that most biomes have evolved strategies to cope with disturbances, as this is part of the adaptation to the prevailing environmental conditions. However, if recent climate change has brought a part of the past biome area to an untypical biome-climate due to rapidly changing climate since 1975, we can expect that the part of the biome that is no longer exposed to the proper biome climate is experiencing more severe and more frequent disturbances than in the past. The same is true for how biome-typical species respond to shifting climate. Yet, a potential biome shift most often translates to a different set of plant functional traits of the biome-dominant species to be optimally adapted. Biome shifts therefore represent a more massive maladaptation than if climate change suggests a shift from one species to another with both species having the same functional properties.
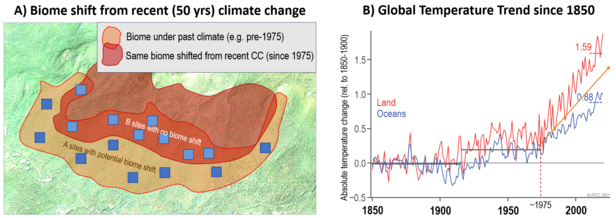
Therefore, we hypothesize that “areas with recent potential biome shift due to ongoing rapid climate change show more severe and more frequent disturbance from abiotic and biotic stress than nearby regions within the same biome but with no potential biome shift” (Fig. 3). Disturbances can originate from decreased or increased precipitation with or without combination with generally raising temperatures. This can enhance drought stress in general, with more severe and longer drought periods, causing diebacks in forest, forest and range fires, or pest outbreaks from weakened dominant species. Yet, it can also enhance biological invasions where warming now favours other functional types, such as upward shifts of tree lines, or the spread of evergreen species into deciduous forest vegetation (termed “laurophilisation”). In this project, we will test this “potential biome shift – stress severity/frequency” hypothesis for different biome transitions. This will allow us to formulate a more general predictive model to forecast what types of disturbances we can expect given the progressing climate change for the different biomes globally.
Analysis steps
- Using continental climate boundaries from part 1f and projecting the potential biome shifts for the last 50 years to map potential shift areas as contrast to those areas per biome that remain unshifted (see Fig. 3).
- Design a sampling protocol to assess biome-level disturbances from remote sensing for regions of potential biome shift and nearby no-shift areas as contrast.
- Assigning the likely type of disturbance from RS-based analyses, literature reviews and fieldwork.
- Testing the hypothesis if disturbance difference between potential shift/no-shift regions.
- Generalizing the potential biome-shift disturbances to future conditions, forecasting where and in what current biome we’d expect what disturbance in the future.
- Focusing on three warm- and cold-temperate (both evergreen and deciduous) forest biomes in more detail including more intense fieldwork to study disturbance processes in more detail.
- Comparing disturbance differences per biome globally by relating continental-scale disturbances to the richness differences and area differences of these biome patches.